利用者:加藤勝憲/非共有相互作用
化学では、非共有結合的相互作用は共有結合とは異なり、電子の共有を伴わず[1]、むしろ分子間や分子内でより分散した電磁相互作用の変化を伴う。非共有結合的相互作用の形成において放出される化学エネルギーは、一般的に1-5 kcal/mol(6.02×1023分子あたり1000-5000カロリー)のオーダーである[2]。非共有結合的相互作用は、静電効果、π効果、ファンデルワールス力、疎水効果などの異なるカテゴリーに分類することができる[3][2]。
非共有結合性相互作用[4]は、タンパク質や核酸のような大きな分子の立体構造を維持する上で重要である。また、非共有結合は、大きな分子が互いに特異的に、しかし一過性に結合する多くの生物学的過程にも関与している(DNAのページの特性の項を参照)。これらの相互作用は、医薬品設計、結晶性、材料の設計、特に自己集合、そして一般的に多くの有機化合物の合成にも大きな影響を与える[3][5][6][7][8]。
crystallinity
非共有結合的相互作用は、同じ分子の異なる部分間(例えばタンパク質フォールディングの際)、あるいは異なる分子間で起こることがあり、したがって分子間力としても議論される。
静電相互作用
[編集]Ionic
[編集]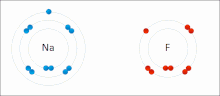
イオン相互作用は、正反対の符号の完全な永久電荷を持つイオンまたは分子の引力を伴う。例えば、フッ化ナトリウムは、ナトリウム(Na+)の正電荷とフッ化物(F-)の負電荷が引き合う[9]。しかし、この特殊な相互作用は、水や他の極性の高い溶媒に加えると簡単に壊れてしまう。水中でのイオン対形成は、ほとんどがエントロピー駆動型であり、1つの塩橋は通常、中間のイオン強度Iで約ΔG =5 kJ/molの引力となり、Iがゼロに近くなると値は約8 kJ/molに増加する。ΔG値は通常、相加的であり、遷移金属イオンなどを除き、参加イオンの性質にはほとんど依存しない[10]。
このような相互作用は、特定の原子に局在した電荷を持つ分子にも見られる。例えば、エタノールの共役塩基であるエトキシドに関連する完全な負電荷は、ナトリウムカチオン(Na+)のようなアルカリ金属塩の正電荷を伴うのが一般的である。
atom.
ethoxide, the conjugate base of ethanol, is
alkali metal
cation (Na+).
水素結合
[編集]
水素結合(H-bond)とは、部分的に陽性の水素原子と、電子陰性度が高く、部分的に陰性の酸素原子、窒素原子、硫黄原子、またはフッ素原子(当該水素原子と共有結合していない)との間の双極子-双極子引力を伴う特定のタイプの相互作用である。共有結合ではなく、強い非共有結合の相互作用に分類される。水が室温で液体であり、気体でないのはこのためである(水の分子量が低いため)。一般的に、水素結合の強さは0~4 kcal/molであるが、時には40 kcal/molにもなることがある[3]。
クロロホルムや四塩化炭素のような溶媒中では、例えばアミド間の相互作用で約5kJ/molの付加値が観測される。ライナス・ポーリングによれば、水素結合の強さは本質的に静電荷によって決まる。クロロホルムや四塩化炭素中で何千もの錯体を測定した結果、あらゆる種類のドナーとアクセプターの組み合わせに対して、自由エネルギーの相加的な増加が見られた[11][12]。
In solvents such as chloroform or carbon tetrachloride
Halogen bonding
[編集]
Halogen bonding is a type of non-covalent interaction which does not involve the formation nor breaking of actual bonds, but rather is similar to the dipole–dipole interaction known as hydrogen bonding. In halogen bonding, a halogen atom acts as an electrophile, or electron-seeking species, and forms a weak electrostatic interaction with a nucleophile, or electron-rich species. The nucleophilic agent in these interactions tends to be highly electronegative (such as oxygen, nitrogen, or sulfur), or may be anionic, bearing a negative formal charge. As compared to hydrogen bonding, the halogen atom takes the place of the partially positively charged hydrogen as the electrophile.[要出典]
Halogen bonding should not be confused with halogen–aromatic interactions, as the two are related but differ by definition. Halogen–aromatic interactions involve an electron-rich aromatic π-cloud as a nucleophile; halogen bonding is restricted to monatomic nucleophiles.[5]
Van der Waals forces
[編集]Van der Waals forces are a subset of electrostatic interactions involving permanent or induced dipoles (or multipoles). These include the following:
- permanent dipole–dipole interactions, alternatively called the Keesom force
- dipole-induced dipole interactions, or the Debye force
- induced dipole-induced dipole interactions, commonly referred to as London dispersion forces
Hydrogen bonding and halogen bonding are typically not classified as Van der Waals forces.
Dipole–dipole
[編集]
Dipole-dipole interactions are electrostatic interactions between permanent dipoles in molecules. These interactions tend to align the molecules to increase attraction (reducing potential energy). Normally, dipoles are associated with electronegative atoms, including oxygen, nitrogen, sulfur, and fluorine.
例えば、マニキュアの除光液の有効成分であるアセトンは、カルボニルと正味の双極子を持っている(図2参照)。酸素は共有結合している炭素よりも電気陰性度が高いため、その結合に関連する電子は炭素よりも酸素に近づき、酸素には部分的な負電荷(δ-)が、炭素には部分的な正電荷(δ+)が生じる。電子はまだ酸素と炭素の共有結合によって共有されているので、これらは完全な電荷ではない。もし電子の共有がなくなれば、酸素と炭素の結合は静電相互作用となる。
- H δ + − Cl δ − ⋯ H δ + − Cl δ − {\displaystyle {\overset {\color {Red}\delta +}{{\ce {H}}}}-{\overset {\color {Red}\delta -}{{\ce {Cl}}}}\cdots {\overset {\color {Red}\delta +}{{\ce {H}}}}-{\overset {\color {Red}\delta -}{{\ce {Cl}}}}}
Often molecules contain dipolar groups, but have no overall dipole moment. This occurs if there is symmetry within the molecule that causes the dipoles to cancel each other out. This occurs in molecules such as tetrachloromethane. Note that the dipole-dipole interaction between two individual atoms is usually zero, since atoms rarely carry a permanent dipole. See atomic dipoles.
Dipole-induced dipole
[編集]A dipole-induced dipole interaction (Debye force) is due to the approach of a molecule with a permanent dipole to another non-polar molecule with no permanent dipole. This approach causes the electrons of the non-polar molecule to be polarized toward or away from the dipole (or "induce" a dipole) of the approaching molecule.[13] Specifically, the dipole can cause electrostatic attraction or repulsion of the electrons from the non-polar molecule, depending on orientation of the incoming dipole.[13] Atoms with larger atomic radii are considered more "polarizable" and therefore experience greater attractions as a result of the Debye force.[要出典]
London dispersion forces
[編集]London dispersion forces[14][15][16][17] are the weakest type of non-covalent interaction. In organic molecules, however, the multitude of contacts can lead to larger contributions, particularly in the presence of heteroatoms. They are also known as "induced dipole-induced dipole interactions" and present between all molecules, even those which inherently do not have permanent dipoles. Dispersive interactions increase with the polarizability of interacting groups, but are weakened by solvents of increased polarizability.[18] They are caused by the temporary repulsion of electrons away from the electrons of a neighboring molecule, leading to a partially positive dipole on one molecule and a partially negative dipole on another molecule.[6] Hexane is a good example of a molecule with no polarity or highly electronegative atoms, yet is a liquid at room temperature due mainly to London dispersion forces. In this example, when one hexane molecule approaches another, a temporary, weak partially negative dipole on the incoming hexane can polarize the electron cloud of another, causing a partially positive dipole on that hexane molecule. In absence of solvents hydrocarbons such as hexane form crystals due to dispersive forces ; the sublimation heat of crystals is a measure of the dispersive interaction. While these interactions are short-lived and very weak, they can be responsible for why certain non-polar molecules are liquids at room temperature.
π-effects
[編集]π-effects can be broken down into numerous categories, including π-π interactions, cation-π & anion-π interactions, and polar-π interactions. In general, π-effects are associated with the interactions of molecules with the π-systems of conjugated molecules such as benzene.[3]
π–π interaction
[編集]
π–π interactions are associated with the interaction between the π-orbitals of a molecular system.[3] The high polarizability of aromatic rings lead to dispersive interactions as major contribution to so-called stacking effects. These play a major role for interactions of nucleobases e.g. in DNA.[19] For a simple example, a benzene ring, with its fully conjugated π cloud, will interact in two major ways (and one minor way) with a neighboring benzene ring through a π–π interaction (see figure 3). The two major ways that benzene stacks are edge-to-face, with an enthalpy of ~2 kcal/mol, and displaced (or slip stacked), with an enthalpy of ~2.3 kcal/mol.[3] The sandwich configuration is not nearly as stable of an interaction as the previously two mentioned due to high electrostatic repulsion of the electrons in the π orbitals.[3]
Cation–π and anion–π interaction
[編集]
Cation–pi interactions involve the positive charge of a cation interacting with the electrons in a π-system of a molecule.[3] This interaction is surprisingly strong (as strong or stronger than H-bonding in some contexts),[3] and has many potential applications in chemical sensors.[20] For example, the sodium ion can easily sit atop the π cloud of a benzene molecule, with C6 symmetry (See figure 4).
Anion–π interactions are very similar to cation–π interactions, but reversed. In this case, an anion sits atop an electron-poor π-system, usually established by the placement of electron-withdrawing substituents on the conjugated molecule[21]

Polar–π
[編集]Polar–π interactions involve molecules with permanent dipoles (such as water) interacting with the quadrupole moment of a π-system (such as that in benzene (see figure 5). While not as strong as a cation-π interaction, these interactions can be quite strong (~1-2 kcal/mol), and are commonly involved in protein folding and crystallinity of solids containing both hydrogen bonding and π-systems.[3] In fact, any molecule with a hydrogen bond donor (hydrogen bound to a highly electronegative atom) will have favorable electrostatic interactions with the electron-rich π-system of a conjugated molecule.[要出典]
Hydrophobic effect
[編集]The hydrophobic effect is the desire for non-polar molecules to aggregate in aqueous solutions in order to separate from water.[22] This phenomenon leads to minimum exposed surface area of non-polar molecules to the polar water molecules (typically spherical droplets), and is commonly used in biochemistry to study protein folding and other various biological phenomenon.[22] The effect is also commonly seen when mixing various oils (including cooking oil) and water. Over time, oil sitting on top of water will begin to aggregate into large flattened spheres from smaller droplets, eventually leading to a film of all oil sitting atop a pool of water. However the hydrophobic effect is not considered a non-covalent interaction as it is a function of entropy and not a specific interaction between two molecules, usually characterized by entropy.enthalpy compensation.[23][24][25] An essentially enthalpic hydrophobic effect materializes if a limited number of water molecules are restricted within a cavity; displacement of such water molecules by a ligand frees the water molecules which then in the bulk water enjoy a maximum of hydrogen bonds close to four.[26][27]
Examples
[編集]Drug design
[編集]Most pharmaceutical drugs are small molecules which elicit a physiological response by "binding" to enzymes or receptors, causing an increase or decrease in the enzyme's ability to function. The binding of a small molecule to a protein is governed by a combination of steric, or spatial considerations, in addition to various non-covalent interactions, although some drugs do covalently modify an active site (see irreversible inhibitors). Using the "lock and key model" of enzyme binding, a drug (key) must be of roughly the proper dimensions to fit the enzyme's binding site (lock).[28] Using the appropriately sized molecular scaffold, drugs must also interact with the enzyme non-covalently in order to maximize binding affinity binding constant and reduce the ability of the drug to dissociate from the binding site. This is achieved by forming various non-covalent interactions between the small molecule and amino acids in the binding site, including: hydrogen bonding, electrostatic interactions, pi stacking, van der Waals interactions, and dipole–dipole interactions.
Non-covalent metallo drugs have been developed. For example, dinuclear triple-helical compounds in which three ligand strands wrap around two metals, resulting in a roughly cylindrical tetracation have been prepared. These compounds bind to the less-common nucleic acid structures, such as duplex DNA, Y-shaped fork structures and 4-way junctions.[29]
Protein folding and structure
[編集]The folding of proteins from a primary (linear) sequence of amino acids to a three-dimensional structure is directed by all types of non-covalent interactions, including the hydrophobic forces and formation of intramolecular hydrogen bonds. Three-dimensional structures of proteins, including the secondary and tertiary structures, are stabilized by formation of hydrogen bonds. Through a series of small conformational changes, spatial orientations are modified so as to arrive at the most energetically minimized orientation achievable. The folding of proteins is often facilitated by enzymes known as molecular chaperones.[30] Sterics, bond strain, and angle strain also play major roles in the folding of a protein from its primary sequence to its tertiary structure.
Single tertiary protein structures can also assemble to form protein complexes composed of multiple independently folded subunits. As a whole, this is called a protein's quaternary structure. The quaternary structure is generated by the formation of relatively strong non-covalent interactions, such as hydrogen bonds, between different subunits to generate a functional polymeric enzyme.[31] Some proteins also utilize non-covalent interactions to bind cofactors in the active site during catalysis, however a cofactor can also be covalently attached to an enzyme. Cofactors can be either organic or inorganic molecules which assist in the catalytic mechanism of the active enzyme. The strength with which a cofactor is bound to an enzyme may vary greatly; non-covalently bound cofactors are typically anchored by hydrogen bonds or electrostatic interactions.
Boiling points
[編集]Non-covalent interactions have a significant effect on the boiling point of a liquid. Boiling point is defined as the temperature at which the vapor pressure of a liquid is equal to the pressure surrounding the liquid. More simply, it is the temperature at which a liquid becomes a gas. As one might expect, the stronger the non-covalent interactions present for a substance, the higher its boiling point. For example, consider three compounds of similar chemical composition: sodium n-butoxide (C4H9ONa), diethyl ether (C4H10O), and n-butanol (C4H9OH).

The predominant non-covalent interactions associated with each species in solution are listed in the above figure. As previously discussed, ionic interactions require considerably more energy to break than hydrogen bonds, which in turn are require more energy than dipole–dipole interactions. The trends observed in their boiling points (figure 8) shows exactly the correlation expected, where sodium n-butoxide requires significantly more heat energy (higher temperature) to boil than n-butanol, which boils at a much higher temperature than diethyl ether. The heat energy required for a compound to change from liquid to gas is associated with the energy required to break the intermolecular forces each molecule experiences in its liquid state.
References
[編集]- ^ “Glossary”. Molecular Cell Biology (4th ed.). New York: W.H. Freeman. (2000). ISBN 978-0-7167-3136-8
- ^ a b “Noncovalent bonds”. Molecular Cell Biology (4th ed.). New York: W.H. Freeman. (2000). ISBN 978-0-7167-3136-8
- ^ a b c d e f g h i j Modern Physical Organic Chemistry. Sausalito, CA: University Science. (2004). ISBN 978-1-891389-31-3
- ^ “Introduction”. Analytical Methods in Supramolecular Chemistry (2nd ed.). Wiley. (March 2012). ISBN 978-3-527-32982-3
- ^ a b “Chemical double-mutant cycles: dissecting non-covalent interactions”. Chemical Society Reviews 36 (2): 172–188. (February 2007). doi:10.1039/b603842p. PMID 17264921.
- ^ a b Chemistry: The Central Science (11th ed.). Upper Saddle River, NJ: Pearson Prentice Hall. (2009). ISBN 978-0-13-600617-6
- ^ “Self-Assembly”. Encyclopedia of nanoscience and society. Thousand Oaks, Calif.: Sage. (2010). doi:10.4135/9781412972093.n412. ISBN 978-1-4129-7209-3
- ^ “Experimental Binding Energies in Supramolecular Complexes”. Chemical Reviews 116 (9): 5216–5300. (May 2016). doi:10.1021/acs.chemrev.5b00583. PMID 27136957.
- ^ Ionic Interactions in Natural and Synthetic Macromolecules. John Wiley & Sons. (2012). ISBN 978-0-470-52927-0
- ^ “Binding mechanisms in supramolecular complexes”. Angewandte Chemie 48 (22): 3924–3977. (2009). doi:10.1002/anie.200802947. PMID 19415701.
- ^ “Scales of Solute Hydrogen-bonding: their Construction and Application to Physicochemical and Biochemical Processes”. Chem. Soc. Rev. 22 (2): 73–83. (1993). doi:10.1039/CS9932200073.
- ^ “Quantifying hydrogen bonding in QSAR and molecular modeling”. SAR and QSAR in Environmental Research 16 (3): 287–300. (June 2005). doi:10.1080/10659360500036893. PMID 15804815.
- ^ a b “Induced-Dipole Forces”. 11 November 2013閲覧。
- ^ Noncovalent Forces. Springer. (2015). ISBN 978-3-319-14162-6
- ^ Non-covalent Interactions in Quantum Chemistry and Physics: Theory and Applications. Elsevier. (2017). ISBN 978-0-12-809835-6
- ^ Non-covalent Interactions in the Synthesis and Design of New Compounds. Wiley. (2016). ISBN 978-1-119-10989-1
- ^ Non-Covalent Interactions: Theory and Experiment. Theoretical and Computational Chemistry Series. Royal Society of Chemistry. (2009). ISBN 978-1-84755-853-4
- ^ “Dispersive interactions in solution complexes”. Accounts of Chemical Research 48 (7): 1815–1822. (July 2015). doi:10.1021/acs.accounts.5b00111. PMID 26083908.
- ^ “On the importance and origin of aromatic interactions in chemistry and biodisciplines”. Accounts of Chemical Research 46 (4): 927–936. (April 2013). doi:10.1021/ar300083h. PMID 22872015.
- ^ “Cation-π interaction: its role and relevance in chemistry, biology, and material science”. Chemical Reviews 113 (3): 2100–2138. (March 2013). doi:10.1021/cr300222d. PMID 23145968.
- ^ “Anion-pi Interactions: do they exist?”. Angewandte Chemie 41 (18): 3389–3392. (September 2002). doi:10.1002/1521-3773(20020916)41:18<3389::AID-ANIE3389>3.0.CO;2-S. PMID 12298041.
- ^ a b IUPAC (2009). “Hydrophobic interaction”. Compendium of Chemical Terminology. doi:10.1351/goldbook.H02907. ISBN 978-0-9678550-9-7 11 November 2013閲覧。
- ^ “The hydrophobic effect”. Curr. Opinion Coll. Interface Sci. 22: 14–22. (2016). doi:10.1016/j.cocis.2016.02.001.
- ^ “Molecular Shape and the Hydrophobic Effect”. Annual Review of Physical Chemistry 67: 307–329. (May 2016). Bibcode: 2016ARPC...67..307H. doi:10.1146/annurev-physchem-040215-112316. PMC 5571648. PMID 27215816 .
- ^ “Water-Mediated Hydrophobic Interactions”. Annual Review of Physical Chemistry 67: 617–638. (May 2016). Bibcode: 2016ARPC...67..617B. doi:10.1146/annurev-physchem-040215-112412. PMID 27215821.
- ^ “Is it the shape of the cavity, or the shape of the water in the cavity?”. The European Physical Journal Special Topics 223 (5): 853–891. (2014). Bibcode: 2014EPJST.223..853S. doi:10.1140/epjst/e2013-01818-y .
- ^ “The hydrophobic effect revisited--studies with supramolecular complexes imply high-energy water as a noncovalent driving force”. Angewandte Chemie 53 (42): 11158–11171. (October 2014). doi:10.1002/anie.201310958. PMID 25070083.
- ^ “Biomolecules: Enzymes”. ChemPages Netorials. University of Wisconsin - Madison. 27 October 2013閲覧。
- ^ “Non-covalent Metallo-Drugs: Using Shape to Target DNA and RNA Junctions and Other Nucleic Acid Structures”. Metal Ions in Life Sciences (de Gruyter GmbH) 18: 303–324. (February 2018). doi:10.1515/9783110470734-017. ISBN 978-3-11-047073-4. PMID 29394030.
- ^ Biochemistry (4th ed.). Hoboken, NJ: John Wiley & Sons. (2010). ISBN 978-0-470-57095-1
- ^ The organic chemistry of drug design and drug action (2. ed.). Amsterdam [u.a.]: Elsevier. (2004). ISBN 978-0-12-643732-4
[[Category:超分子]] [[Category:化学結合]] [[Category:未査読の翻訳があるページ]]